Non-Ig derived alternatives
The common characteristic for antibody mimetics is the intention to provide alternatives that perform similar or better than antibodies. Their origins are very diverse and accordingly there is a wide range of structurally very heterogenous platforms available. Generally, these alternatives consist of short polypeptide or oligonucleotide chains that assume a simple, thermodynamically stable fold. They must tolerate amino acid or nucleotide substitutions within their target binding regions in order to be amenable to screening of combinatorial libraries.
Many of these antibody mimetics are devoid of disulfide bonds and can therefore be produced in the reducing conditions of the cytoplasm. This and the absence of posttranslational modifications widens the range of available expression systems, including inexpensive bacterial systems. In addition, increased reproducibility and the ability to develop binders to difficult targets are among the key benefits of non-Ig derived antibody alternatives.
Besides production, storage is also often easier for these products than for antibodies, as they retain stability under conditions that would denature or damage conventional antibodies. To this end, antibody alternatives rely on a biologically inert scaffold that is conducive to the formation of structures in order to provide a large interaction surface for tight binding with modifiable patches that establish the specific contacts to the respective target with affinities oftentimes in the nM- to pM-range. The repeats should be self-organizing, ideally without the need for disulfide bridges. Structural residues should be different from those establishing specificity in order to allow for straightforward engineering of the protein’s specificity without jeopardizing its structural integrity.
Furthermore, their aptness for engineering stems also from the fact that these scaffolds for specific binders offer a way to circumvent intellectual property. Antibody based drugs are extensively protected by patents, making it difficult for drug companies to broaden their use. Due to their small size some antibody mimetics must be fused to a carrier such as IgG to slow down renal clearance and achieve the desired pharmacokinetic properties.
Non-Ig alternatives are not yet being used on the same scale as conventional immunoglobulins.That may very well change in the near future, both for clinical and research applications. There is a plethora of naturally existing protein domains available for engineering of specific binders, such as:
Aptamers
Aptamers are nucleic acid or peptide based binders that specifically recognized proteins, nucleic acids, and other molecules. Whole cells and even tissues are also among the targets. Nucleic acid aptamers are engineered through SELEX or in vitro selection. X-aptamers incorporate chemically modified nucleotides and DNA backbones to achieve a greater range of possible targets or increase affinity and specificity. However, for the same reason it is not possible to apply the same amplification and selection mechanisms as for RNA- and DNA-aptamers. In contrast, peptide based aptamers consist of a variable loop region that is attached on both ends to a large scaffold protein for solubility.
Traditionally, a randomized peptide expression library is displayed by the E. coli thioredoxin A (TrxA) protein. Other scaffolds such as GFP have also been used. Aptamers with the desired specificity are selected using the yeast two hybrid system or established display techniques.
Slow Off-rate Modified Aptamers (SOMAmers1) are a particular class of aptamers that consist of a short DNA sequence that incorporate several modified bases with protein-like side chains. Hence, they exploit the potential of synthetic nucleotide modifications to confer protein like characteristics and diversity to a nucleic acid scaffold.
DARPins2
Designed ankyrin repeat proteins are based on the structure of naturally occurring ankyrins, a class of protein that mediates the attachment of integral membrane proteins to the membrane cytoskeleton. They are composed typically of four to six 33 aa long repeats consisting of a β-turns followed by two antiparallel α-helices.
Retaining structurally important, conserved residues as a scaffold, DARPins are very amenable to engineering of the residues that contact the binding partner by ribosome or phage display methods.
The modules are held together by hydrophobic interaction except for the hydrophilic N- and C-termini (or caps) exposed to the solvent. Due to strong coupling between the repeats DARPins with at least four internal repeats highly are resistant against denaturation and they express well in E. coli as well as cell free expression systems.
Affimers
Rational design of a novel scaffold for the presentation of constrained peptides based on the desired characteristics for antibody mimetics lead to the development of the human stefin A (SteA) based affimers. Their stefin A triple mutant (STM) consists of 98 aa in a single chain and without any posttranslational modifications3. Furthermore, the stability of the fold is unaffected by insertion of a peptide that confers target specificity to the protein. These STM based affimers are considered type-I affimers.
Type-II affimers are based on the cysteine protease inhibitor cystatin4. Here, cystatin serves as a scaffold to display two loops and a variable N-terminal section that confer specificity to the protein. The two loops can extend over 12 to 36 aa each, thus creating alarge interface for interaction with the targeted antigen. The extent of this interface is responsible for the high specificity and binding affinity
Avimers5
Avidity multimers are derived from human A-domains that are present in various cell surface receptors. They are formed by two or more peptide sequences of 30-35 aa, each of which recognizes a different epitope, thus forming proteins that can bind to multiple sites on one or several targets with down to picomolar affinities. The different domains are generated by exon shuffling and sequential selections.
The structure of each ‘monomer’ is defined by four calcium binding residues, two scaffold residues and six cysteines forming three disulfide bridges. Remarkably, these disulfide bridges can be successfully formed upon oxidization and the subunits properly folded after production in the cytoplasm of E. coli.
Knottins6
Knottins are small disulfide rich proteins with at least three disulfide bridges characterized by a trefoil disulfide through disulfide knot. This core structure renders them very resistant to proteolysis and thermal changes while exposed loops confer specificity towards the target of interest. Owing to their small size of only a few kDa knottins have considerable potential as drugs and novel molecular imaging reagents.
Monobodies7
In spite of their name, monobodies are not antibody derivatives. They use instead the type III domain of human Fibronectin (FN3) as a framework onto which loops for specific target recognition can be grafted. The FN3 scaffold comprises 94aa and resembles the antibody VH domain. It does however not contain any disulfide bridges. Its binding specificity can readily be altered using phage and yeast display techniques.
Affinity clamps8
These recombinant binding proteins combine two protein domains that are connected via a hinge region and that “clamp” down on short peptide motifs. Both domains work synergistically to reach levels of specificity and affinity that could not be attained by either of the two domains separately. Their specificity is in fact high enough to capture a single peptide target in the cellular background. Specificity and affinity of the interaction domain and the enhancer domain can be altered by iterative mutation and selection cycles.
Conclusion
This list can only provide a glimpse of the diverse platforms that are available now as antibody mimetics. It is far from being comprehensive and omits even some mature scaffolds such as affilins9, protein A derived affibodies 10, and the lipocalin related anticalins11.
Presently, just a few of these platforms have given rise to specific binders that are readily available for research applications. However, research and development efforts on these various antibody alternatives was basically limited to the last 25 years. One can only imagine the possibilities that will open up with these molecules both as therapeutics and research reagents in the next 100 years.
Additional Information
Conventional immunoglobulin alternatives (Part 1)
References
- Unique motifs and hydrophobic interactions shape the binding of modified DNA ligands to protein targets." in: Proceedings of the National Academy of Sciences of the United States of America, Vol. 109, Issue 49, pp. 19971-6, (2013) (PubMed). : "
- High-affinity binders selected from designed ankyrin repeat protein libraries." in: Nature biotechnology, Vol. 22, Issue 5, pp. 575-82, (2004) (PubMed). : "
- Design and validation of a neutral protein scaffold for the presentation of peptide aptamers." in: Journal of molecular biology, Vol. 352, Issue 5, pp. 1118-33, (2005) (PubMed). : "
- Adhiron: a stable and versatile peptide display scaffold for molecular recognition applications." in: Protein engineering, design & selection : PEDS, Vol. 27, Issue 5, pp. 145-55, (2015) (PubMed). : "
- Multivalent avimer proteins evolved by exon shuffling of a family of human receptor domains." in: Nature biotechnology, Vol. 23, Issue 12, pp. 1556-61, (2006) (PubMed). : "
- The KNOTTIN website and database: a new information system dedicated to the knottin scaffold." in: Nucleic acids research, Vol. 32, Issue Database issue, pp. D156-9, (2004) (PubMed). : "
- The fibronectin type III domain as a scaffold for novel binding proteins." in: Journal of molecular biology, Vol. 284, Issue 4, pp. 1141-51, (1999) (PubMed). : "
- Generation of high-performance binding proteins for peptide motifs by affinity clamping." in: Methods in enzymology, Vol. 523, pp. 285-302, (2013) (PubMed). : "
- Affilin-novel binding molecules based on human gamma-B-crystallin, an all beta-sheet protein." in: Journal of molecular biology, Vol. 372, Issue 1, pp. 172-85, (2007) (PubMed). : "
- A combinatorial library of an alpha-helical bacterial receptor domain." in: Protein engineering, Vol. 8, Issue 6, pp. 601-8, (1996) (PubMed). : "
- Small antibody-like proteins with prescribed ligand specificities derived from the lipocalin fold." in: Proceedings of the National Academy of Sciences of the United States of America, Vol. 96, Issue 5, pp. 1898-903, (1999) (PubMed). : "
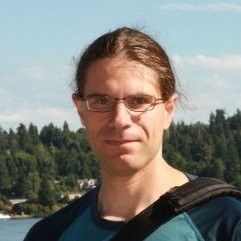
Goal-oriented, time line driven scientist, proficiently trained in different academic institutions in Germany, France and the USA. Experienced in the life sciences e-commerce environment with a focus on product development and customer relation management.
Go to author page