Hallmarks of Ageing
The inevitability of aging has fascinated humanity since antiquity. Despite remarkable scientific advances, the complexity of aging continues to impede a complete understanding. In 2013, the Hallmarks of Aging were introduced by López-Otín et al. to establish an organized, systematic, and integrative framework for research in this field. More than a decade later, developments in the field necessitated an updated version with three additional hallmarks. This review aims to explain the underlying biological mechanisms, highlight recent insights and emerging key players, and provide high-quality antibodies for enabling further research on these essential antigens.
Fig. 1: Hallmarks of Ageing based on López-Otín et al.. Click on a hallmark for further information.
Aging hallmarks have been categorized into three types: primary, antagonistic, and integrative hallmarks. Primary hallmarks directly cause damage onset and continuously have harmful effects. Antagonistic hallmarks are context-dependent with protective effects at moderate levels but harmful effects when hyperactivated. Integrative hallmarks occur when the body's compensatory mechanisms are unable to maintain tissue homeostasis under the accumulated damage.
The interconnected nature of ageing hallmarks implies that experimentally enhancing or suppressing one hallmark often influences others, highlighting the systemic and multifactorial nature of the ageing process. Therefore, ageing must be understood as a linked system. Each hallmark is not only a manifestation of ageing but also a potential entry point for investigating its underlying mechanisms and for developing targeted anti-ageing therapeutic strategies.
Hallmarks of Ageing: Definition
Based on the “The hallmarks of cancer” by Hanahan and Weinberg, López-Otín et al. established three criteria that a biological process must fulfil to be considered as a hallmark of aging: it has to appear during physiological aging, it has to accelerate aging when aggravated experimentally, and its experimental alleviation has to slow aging, thus increasing healthy lifespan.
Genome Instability
Genome instability belongs to the primary hallmarks of ageing. It refers to an increased tendency for alterations in the genome, like mutations and chromosomal rearrangement. As organisms age, several cellular processes that maintain genomic integrity become impaired, including DNA damage recognition (ATM) and DNA repair pathways like homologous recombination and non-homologous end joining (ATR, BRCA1). The result is a higher burden of DNA lesions and mutations in somatic cells, which not only contributes to cellular senescence and functional decline but also increases the risk of malignant transformation. Therefore, genome instability is also a hallmark of cancer. Accumulated mutations over time can inactivate tumor suppressor genes (TP53) or activate oncogenes.
Telomere Attrition
Telomeres are repetitive nucleotide sequences (TTAGGG repeats in humans) located at the ends of linear chromosomes, serving to protect chromosomal DNA from deterioration or fusion with neighboring chromosomes. Telomere attrition is a cumulative process driven over time by replicative cell divisions (mitotic clock), oxidative stress or inflammatory environments. Telomeres are protected by shelterin, a specialized multi-protein complex that binds specifically to telomeric DNA, protecting chromosome ends and regulating telomere maintenance.
With each round of DNA replication, telomeres progressively shorten in somatic cells, unless compensated by telomerase activity. Critically shortened telomeres lose their protective function. They have fewer binding sites for TRF1 and TRF2, leading to partial or complete dissociation of shelterin components.
Reprogramming Rejuvenation Strategies
Genetic reprogramming-induced rejuvenation introduces specific transcription factors (such as Yamanaka factors: Oct4, Sox2, Klf4, and c-Myc) into somatic cells to revert them to a more youthful or pluripotent state. This process resets epigenetic markers associated with aging and can partially reverse cellular aging without fully inducing pluripotency.
Chemical reprogramming-induced rejuvenation, by contrast, uses small molecules or drug-like compounds to modulate signaling pathways and epigenetic regulators to achieve similar rejuvenating effects without genetic manipulation. These compounds can induce a youthful gene expression profile and reverse aging markers with potentially lower risk and greater controllability.
Epigenetic Alterations
Epigenetic mechanisms emerged as key contributors to the alterations of genome structure and function that accompany aging. The three pillars of epigenetic regulation are DNA methylation, histone modifications, and noncoding RNA species. Alterations of these epigenetic mechanisms affect the vast majority of nuclear processes, including gene transcription and silencing, DNA replication and repair, cell cycle progression, and telomere and centromere structure and function. Transcription factors can induce cellular age reversal though epigenetic reprogramming.
The Circadian Clock
The circadian clock is an intrinsic time-keeping system that regulates daily physiological and metabolic rhythms in alignment with the 24-hour day-night cycle. Disruption of circadian rhythms contributes to age-related decline in cellular function, increased inflammation, and impaired tissue regeneration. Aging is associated with dampened circadian gene expression and reduced amplitude of clock-controlled biological processes, which may accelerate functional deterioration and disease susceptibility.
BMAL1 (Brain and Muscle ARNT-Like 1): A transcription factor that forms a heterodimer with CLOCK to activate the transcription of circadian target genes, including those involved in metabolism, DNA repair, and oxidative stress response. BMAL1 is essential for maintaining circadian rhythm and its deficiency is linked to premature ageing phenotypes. PER2 (Period Circadian Regulator 2): Part of the negative feedback loop that inhibits the activity of the BMAL1:CLOCK complex. PER2 helps regulate the timing and robustness of the circadian cycle. Dysregulation of PER2 has been associated with disrupted sleep patterns and age-related disorders.
Loss of Proteostasis
Loss of proteostasis is a critical hallmark of ageing due to its central role in maintaining protein quality control, which is essential for cellular function and organismal health. Proteostasis involves a tightly regulated network of molecular chaperones, proteolytic systems (e.g., the ubiquitin-proteasome system and autophagy-lysosome pathway), and stress response pathways that collectively ensure proper protein folding, prevent aggregation, and mediate the degradation of damaged or misfolded proteins.
With age, this proteostasis network becomes progressively impaired, leading to the accumulation of dysfunctional proteins and aggregates that can disrupt cellular homeostasis. This proteotoxic stress contributes to cellular senescence, impaired stress responses, and chronic inflammation, and is implicated in the pathogenesis of various age-associated diseases, particularly neurodegenerative disorders such as Alzheimer’s, Parkinson’s, and Huntington’s diseases. Thus, the decline in proteostasis is not only a marker of cellular ageing but also a driver of systemic functional decline across tissues.
Disabled Macroautophagy
With advancing age, macroautophagy becomes progressively compromised. Autophagosome formation is reduced, lysosomal degradation only functions inefficient and expression or activity of key autophagy-related proteins is altered. This decline compromises the cell’s ability to clear damaged organelles and aggregated proteins, contributing to cellular dysfunction and age-related pathologies.
LC3 plays a central role in autophagosome biogenesis. During autophagy, cytosolic LC3-I is lipidated to form LC3-II, which associates with the autophagosomal membrane and is widely used as a marker of autophagosome formation. In ageing cells, reduced LC3-II levels often reflect impaired autophagosome maturation or increased turnover due to defective lysosomal fusion. p62/SQSTM1 functions as a selective autophagy receptor that binds ubiquitinated cargo and delivers it to autophagosomes via LC3 interaction. Under normal conditions, p62 is degraded during autophagy. In aged cells, its accumulation indicates impaired autophagic flux and serves as a marker of dysfunctional cargo clearance.
The antagonistic hallmarks exert dual effects depending on their activity levels—being beneficial at low or transient levels but becoming harmful when chronically activated or excessively intensified, as typically observed with advancing age. Among the initially proposed hallmarks of ageing, deregulated nutrient sensing, mitochondrial dysfunction, and cellular senescence are classified within this category.
Deregulated Nutrient Sensing
Deregulated nutrient-sensing occurs when the cell mechanisms for determining the presence or absence of nutrients fail, so instead of protecting against nutrient scarcity they produce deleterious effects. With advancing age, the IGF1 and mTOR pathways often become hyperactivated, promoting anabolic processes and inhibiting autophagy, which contributes to cellular damage and reduced stress resistance. Conversely, longevity-associated pathways such as AMPK and sirtuins, which support catabolic metabolism and stress resilience, are often downregulated. On the other hand, inhibition of MTORC1 complex, a master regulator of nutrient-sensing mechanisms, increases lifespan in model organisms.
Mitochondrial Dysfunction
Mitochondrial dysfunction in ageing arises from cumulative damage to mitochondrial DNA, impaired electron transport chain (ETC) function, and altered dynamics of mitochondrial biogenesis and mitophagy. These defects lead to reduced ATP production, increased production of reactive oxygen species (ROS), and disruption of redox homeostasis.
These harmful species, together with the decay in the mitochondrial function, can promote the permeabilization of the mitochondria, causing inflammation and cell death. However, when this occurs only at a low-grade during youth, it triggers what is called “mitohormesis”, which promotes cell survival and adaptation to stress.
Mitohormesis
Mitohormesis refers to a biological concept in which mild mitochondrial stress induces an adaptive cellular response that ultimately promotes health and longevity. Specifically, low levels of mitochondrial ROS, traditionally considered damaging, can serve as signaling molecules that activate protective pathways such as antioxidant defense systems, mitochondrial biogenesis, and autophagy.
This adaptive response enhances cellular resilience, improves metabolic homeostasis, and may delay the onset of age-related dysfunction. Importantly, mitohormesis underscores the idea that not all ROS are deleterious—in controlled amounts, they are essential for triggering beneficial stress responses. Thus, mitohormesis offers a mechanistic explanation for why certain interventions, such as caloric restriction or physical exercise, can extend lifespan by transiently increasing mitochondrial stress to induce long-term cellular adaptation.
Cellular Senescence
Cellular senescence is a physiological response to acute or chronic cellular damage. In humans, senescent cells progressively accumulate in various tissues at differing rates, with fibroblasts, endothelial cells, and immune cells being particularly affected. This accumulation is often localized and tissue-specific, and it is associated with a wide range of pathological conditions. Primary senescence can be initiated by diverse forms of cellular stress, including oncogenic signaling, DNA damage, critically shortened telomeres, mitochondrial dysfunction, infection by viruses or bacteria, oxidative stress, nutrient imbalances, and mechanical strain. In contrast, secondary or paracrine senescence arises in response to pro-inflammatory and pro-fibrotic extracellular signals, such as CCL2, IL1β, IL6, IL8, and TGFβ.
A defining hallmark of senescent cells is a stable arrest of cell proliferation, driven by the activation of tumor suppressor pathways involving TP53 and CDKN2A/p16. These pathways act through downstream effectors such as CDKN1A/p21 and the retinoblastoma protein (RB1) family to inhibit cyclin-dependent kinases (CDKs) and E2F transcription factors, thereby halting cell cycle progression.
Cellular senescence is implicated in a range of non-proliferative diseases, including obesity-related metabolic syndrome, type I and type II diabetes, atherosclerosis, and neurodegenerative disorders such as Alzheimer’s and Parkinson’s disease. The pathogenic contribution of senescent cells in these conditions is largely attributed to the senescence-associated secretory phenotype (SASP).
Senescence-Associated Secretory Phenotype
SASP arises from three principal features of senescent cells:
- Transcriptional derepression of endogenous retroelements, particularly LINE-1, leading to the cytosolic accumulation of double-stranded DNA and subsequent activation of the cGAS/STING and Toll-like receptor (TLR) pathways
- Excessive production of reactive oxygen species (ROS) by dysfunctional mitochondria
- Disruption of the autophagy-lysosomal pathway, resulting in increased lysosomal content, which enables histochemical detection via senescence-associated β-galactosidase (SABG) activity.
Given the association of cellular senescence with diverse pathologies, the question is what the biological role of such a cellular reaction could be. While cellular senescence is well established as a mechanism of tumor suppression, there is growing evidence that it also participates in tissue repair. In this context, senescent cells contribute to local fibrosis and secrete factors that recruit immune cells for augmented removal of damaged and senescent cells. Tissue repair can therefore be considered a biphasic process: senescence induction and immune-mediated clearance. Pathological outcomes occur when the second phase fails, leading to persistent accumulation of senescent cells and chronic SASP activity that ultimately promotes fibrosis and tissue dysfunction.
Stem-Cell Exhaustion
Stem cell exhaustion is both a hallmark and a contributor to ageing. Mechanistically, ageing stem cells accumulate DNA damage and telomere shortening, leading to cell cycle arrest or senescence. Epigenetic alterations and mitochondrial dysfunction impair self-renewal and energy homeostasis, while declining proteostasis results in the buildup of misfolded proteins. Additionally, the stem cell niche becomes increasingly inflammatory with age, further compromising stem cell function.
These changes culminate in reduced regenerative capacity across tissues, contributing to age-related dysfunctions such as impaired hematopoiesis, neurodegeneration, and delayed tissue repair. The systemic decline in tissue maintenance not only reflects but also reinforces the ageing phenotype. Understanding and targeting these mechanisms offers promising avenues for therapeutic intervention to preserve stem cell function and extend healthspan.
Cellular Reprogramming
Cellular reprogramming is thought to act across multiple cell types, but its effects on stem and progenitor cells are particularly significant due to their lasting impact. This process involves converting adult somatic cells into induced pluripotent stem cells (iPSCs) using four transcription factors—OCT4, SOX2, KLF4, and MYC (OSKM). Full reprogramming not only shifts cellular identity but also induces rejuvenation, marked by features such as reduced p16 expression, telomere elongation, and resetting of the DNA methylation clock. During this process, cellular aging markers—like DNA damage, epigenetic signatures, and transcriptomic profiles—are reset. The erasure of epigenetic and transcriptional programs likely removes ageing-related alterations. Interestingly, if reprogramming is interrupted, cells can re-differentiate and recover their original identity while retaining the rejuvenated epigenetic and transcriptomic state. This important connection can be exploited to alter and slow down the ageing process.
Cellular Signaling
Ageing is accompanied by progressive disruptions in intercellular communication, increasing systemic noise and impairing both homeostatic balance and adaptive (hormetic) responses. These disruptions manifest as deficits in neural, neuroendocrine, and hormonal signaling—impacting pathways such as adrenergic, dopaminergic, insulin/IGF1, renin-angiotensin, and sex hormone systems, in line with reproductive decline. While these changes are largely driven by intrinsic cellular mechanisms—exemplified by the senescence-associated secretory phenotype (SASP)—they collectively represent a distinct hallmark of ageing. This hallmark bridges cell-autonomous dysfunctions with meta-cellular phenomena, including chronic inflammation, impaired immunosurveillance, and dysregulated host-microbiome interactions, ultimately culminating in dysbiosis.
Fibroaging
Ageing induces progressive damage to long-lived extracellular matrix (ECM) proteins, including the accumulation of advanced glycation end-products (AGEs), carbonylation, carbamylation, elastin fragmentation, and collagen crosslinking. These modifications contribute to increased ECM stiffness and tissue fibrosis—referred to as "fibroaging." This process is driven in part by excessive TGFβ signaling and activation of mechanotransducers such as YAP and TAZ, which promote the expression of pro-fibrotic genes (e.g., transglutaminase-2, lysyl oxidase, and related enzymes).
The stiffened ECM environment also exacerbates cellular senescence, as senescent cells secrete matrix metalloproteinases (MMPs) that further degrade ECM and generate damage-associated molecular patterns (DAMPs), which activate inflammatory and fibrotic pathways. Additionally, increased stiffness enhances WNT signaling, promoting fibroblast activation and expression of pro-fibrotic genes, with extensive crosstalk observed across other pathways such as NOTCH, RAS, TGFβ/SMAD, and hedgehog/GLI. Notably, mechanical stress alone—via the PIEZO1 ion channel—can impair progenitor cell function, such as in oligodendrocyte lineage cells.Experimental models support ECM stiffness as a driver of ageing and highlight therapeutic avenues. Inhibition of PIEZO1 rejuvenates oligodendrocyte progenitors; sustained YAP activity delays ageing by modulating cGAS-STING signaling; and resistance to collagen degradation accelerates vascular ageing. Rare collagen variants (e.g., in COL25A1) may be protective in neurodegeneration. ECM derived from young fibroblasts can reverse features of cellular senescence, and certain ECM molecules (e.g., chondroitin sulfate, hyaluronic acid) have shown lifespan-extending effects in model organisms.
Chronic Inflammation
Chronic low-grade inflammation, or “inflammaging,” is now recognized as a central hallmark of the aging process. It represents a sustained, systemic inflammatory state that emerges even in the absence of infection and progressively undermines physiological resilience, contributing to functional decline and increased disease susceptibility. This persistent inflammatory milieu is not merely a byproduct of aging but a convergent outcome of several underlying hallmarks, including cellular senescence, mitochondrial dysfunction, genomic instability, epigenetic alterations, and impaired autophagy. Clinically, inflammaging manifests both systemically and locally—evident in conditions such as arteriosclerosis, osteoarthritis, neuroinflammation, and intervertebral disc degeneration. Circulating concentrations of inflammatory mediators such as C-reactive protein (CRP), interleukin-6 (IL6), tumor necrosis factor-alpha (TNFα), and IL1β increase with age, serving as biomarkers of inflammaging. Notably, elevated plasma IL-6 levels are strong predictors of all-cause mortality in aging populations. The NLRP3 inflammasome acts as a sensor for cellular damage and mitochondrial stress, driving IL1β release and propagating low-grade inflammation. Concurrently, immune function undergoes a decline marked by shifts in T cell phenotypes: hyperactive pro-inflammatory TH1 and TH17 subsets, reduced immunosurveillance, loss of self-tolerance, and compromised barrier maintenance—all of which fuel systemic inflammation and increase vulnerability to infections, malignancies, and autoimmunity.
The molecular underpinnings of inflammaging are diverse and self-reinforcing. Defective autophagy allows ectopic nuclear and mitochondrial DNA to persist in the cytosol, where it activates innate immune DNA sensors, triggering pro-inflammatory cascades. Genomic instability promotes clonal hematopoiesis of indeterminate potential (CHIP), characterized by expansion of myeloid cells with heightened inflammatory potential, which has been directly linked to cardiovascular aging. In parallel, overexpression of pro-inflammatory proteins arises from epigenetic dysregulation, loss of proteostasis, and hyperactivation of the GH/IGF1/PI3K/AKT/mTORC1 signaling axis. These pathways not only drive cytokine production but also impair tissue repair and regeneration. Additionally, disruptions in circadian rhythms and loss of gut epithelial integrity facilitate microbial translocation and immune activation, further amplifying the inflammatory load. In this complex landscape, inflammation is both a driver and a consequence of multiple aging mechanisms, acting as a central axis through which the biological clock exerts its degenerative influence.
Dysbiosis
Age-related dysbiosis—characterized by reduced microbial diversity, loss of beneficial commensals, and overrepresentation of pro-inflammatory taxa—plays a pivotal role in accelerating ageing processes. This microbial imbalance compromises gut barrier integrity, leading to increased intestinal permeability and systemic translocation of microbial products. Consequently, chronic low-grade inflammation, or "inflammaging," ensues, which is a key contributor to immunosenescence and various age-associated pathologies. The systemic inflammation stemming from dysbiosis adversely affects multiple organ systems. In the central nervous system, it promotes neuroinflammation, contributing to cognitive decline and increasing the risk of neurodegenerative diseases such as Alzheimer's and Parkinson's. Moreover, dysbiosis-induced inflammation is implicated in the pathogenesis of metabolic disorders, cardiovascular diseases, and certain cancers.
The human gut microbiota, once established during early childhood, remains relatively stable throughout most of adulthood. However, aging introduces gradual shifts in its composition and function, ultimately resulting in a decline in microbial diversity and ecological resilience. Studies on centenarians have revealed a reduction in core beneficial taxa such as Bacteroides and Roseburia, alongside an increased abundance of genera like Bifidobacterium and Akkermansia, which are associated with metabolic health and potential pro-longevity effects. Interestingly, in individuals nearing extreme longevity, sustained high levels of Bacteroides and reduced microbial uniqueness have been linked to decreased survival, suggesting that a dynamic and adaptable microbiome may be more advantageous in late life. These age-associated microbial shifts are increasingly recognized as being connected to key geriatric syndromes, including frailty, cognitive decline, depressive symptoms, and heightened systemic inflammation.
Interventions targeting the gut microbiome have shown promise in mitigating age-related decline. For example, supplementation with the probiotic Lactobacillus plantarum GKM3 has been reported to extend lifespan and improve cognitive performance in aging models. Similarly, caloric restriction—an established longevity intervention—reshapes the gut microbiota by enriching beneficial taxa such as Lactobacillus, contributing to healthier aging phenotypes. Age-related increases in gut permeability and inflammation, often driven by the loss of short-chain fatty acid (SCFA)-producing bacteria, can be counteracted by restoring populations of taxa like Akkermansia muciniphila, which improve mucosal barrier integrity and metabolic outcomes. These findings underscore the gut microbiome as both a mediator and modulator of aging, offering a tractable target for interventions aimed at promoting healthspan.
Related Content:
References
- Epigenetic alterations in aging." in: Journal of applied physiology (Bethesda, Md. : 1985), Vol. 109, Issue 2, pp. 586-97, (2015) (PubMed). : "
- The hallmarks of aging." in: Cell, Vol. 153, Issue 6, pp. 1194-217, (2013) (PubMed). : "
- Revisiting the Hallmarks of Aging to Identify Markers of Biological Age." in: The journal of prevention of Alzheimer's disease, Vol. 7, Issue 1, pp. 56-64, (2021) (PubMed). : "
- Hallmarks of aging: An expanding universe." in: Cell, Vol. 186, Issue 2, pp. 243-278, (2023) (PubMed). : "
- Epigenetic regulation of aging: implications for interventions of aging and diseases." in: Signal transduction and targeted therapy, Vol. 7, Issue 1, pp. 374, (2022) (PubMed). : "
- Aging Hallmarks and Progression and Age-Related Diseases: A Landscape View of Research Advancement." in: ACS chemical neuroscience, Vol. 15, Issue 1, pp. 1-30, (2024) (PubMed). : "
- The hallmarks of aging as a conceptual framework for health and longevity research." in: Frontiers in aging, Vol. 5, pp. 1334261, (2024) (PubMed). : "
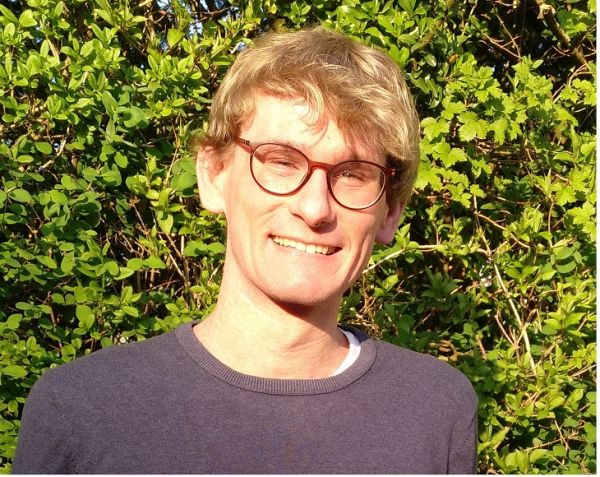
Creative mind of antibodies-online with a keen eye for details. Proficient in the field of life-science with a passion for plant biotechnology and clinical study design. Responsible for illustrated and written content at antibodies-online as well as supervision of the antibodies-online scholarship program.
Go to author page